Phase change and stability
- Sam Stafford (PhD student)
- David Chapman (RA)
- Daniel Eakins
- Simon Bland
Under certain loading conditions, water may undergo a liquid-tosolid phase change during compression, freezing into one of its many phases of ice. Although the Hugoniot of water passes very close to the 'Ice VII' phase at around 3 GPa, the high associated temperatures prevent observation of this phase change under a single shock. Quasi-isentropic compression through either ramped or re-shock experiments can compress water at lower temperatures where it exhibits a slow (~100’s of ns) transformation at approximately 3 GPa, identified as a discontinuity in the velocimetry measurements or through a characteristic darkening observed in high speed photography.
These quasi-isentropic compression experiments have typically used quartz/silica windows to contain the water sample. However, significantly water shows no signs of freezing with sapphire windows until 7 GPa. This suggests that sapphire does not act as the seed for nucleation of the ice. Only under isentropic compression well within the ice VII phase region will sapphire induce a rapid (<100 ns) phase change in water. It is suspected that the water is kept metastable past 3 GPa and reaches its metastable limit at 7 GPa, at which point it undergoes spontaneous volume nucleation.
Using the 100 mm large bore gas gun at Imperial, a multi-cell target containing three individual water samples was subjected to ramp and ring-up loading. These experiments have confirmed that water can undergo freezing during compression to 4 GPa when in contact with fused silica, as evidenced through high-speed imaging and velocimetry. Similar experiments utilising sapphire or silica-coated sapphire windows showed no signs of freezing until compressed past the 7 GPa limit, suggesting freezing is not governed by surface chemistry. Experiments looking into this behaviour are ongoing.
Figure 16: A multi-liquid cell target. The cells have been filled with different coloured dye to verify vacuum sealing.
Figure 17: The same arrested fracture under a scanning electron microscope, showing the void nucleation and coalescence along the transformed band.
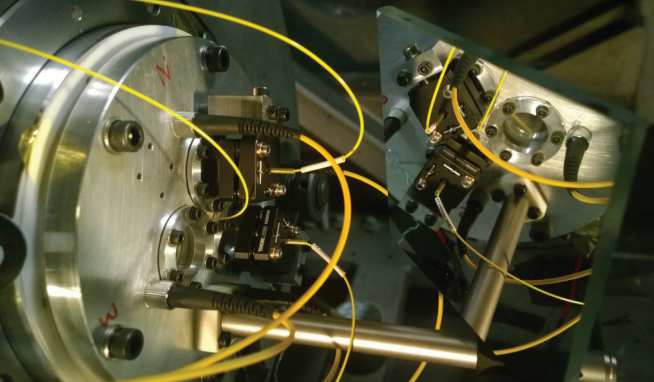
- Ken Watson (PhD student)
- Patricia Hunt
- William Proud
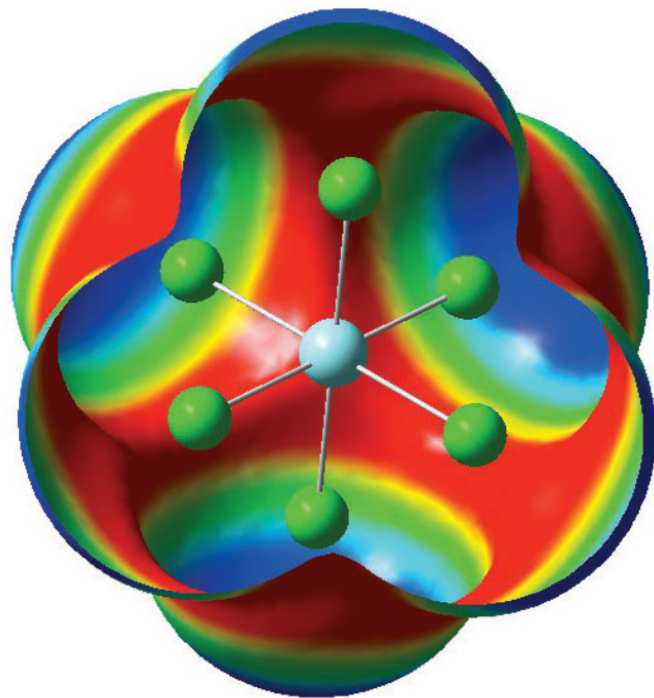
Storage of radioactive material for the past 60 years has led to stockpiles of spent nuclear fuel. Simply storing this material is no longer an option. The spent fuel needs to be separated into its constituent elements which can then be removed or recycled and used to generate power. One method under development is the pyroprocess. Part of the pyroprocess involves dissolving the spent fuel rods in an electrorefiner containing molten chloride salts. Different species form as the metals interact with the solvent ions, leading to changes in the chemistry of the melt. This project aims to use a combination of computational modelling and experimental work to investigate the species formed and to study how these ions interact with the molten solvent. This combination of techniques allows for experimental studies on non-radioactive elements to validate computational models which can then be used to explore the actinide elements. The knowledge gained will be used in the further development of pyroprocesses and for a more effective separation of radioactive elements.
Figure 29: The computed electrostatic potential (ESP) around [YCl6]3-. Areas in blue show a positive potential while the red areas show a negative potential.