Semileptonic decays
Due to their high branching fractions and relatively clean theoretical predictions, semileptonic decays are an excellent tool for testing the SM with high precision. In particular they can be used to extract the elements of the CKM matrix, which are fundamental parameters of the SM. They may also be used to search for new physics by comparing measurements with theory predictions. Over the last ten years there have been consistent hints of lepton universality violation in semileptonic decays. If these discrepancies are confirmed it would be evidence of physics beyond the SM. These observables, R(D) and R(D*), which compare decays to final states with τ leptons or light leptons, currently show a deviation from the SM of around three standard deviations.
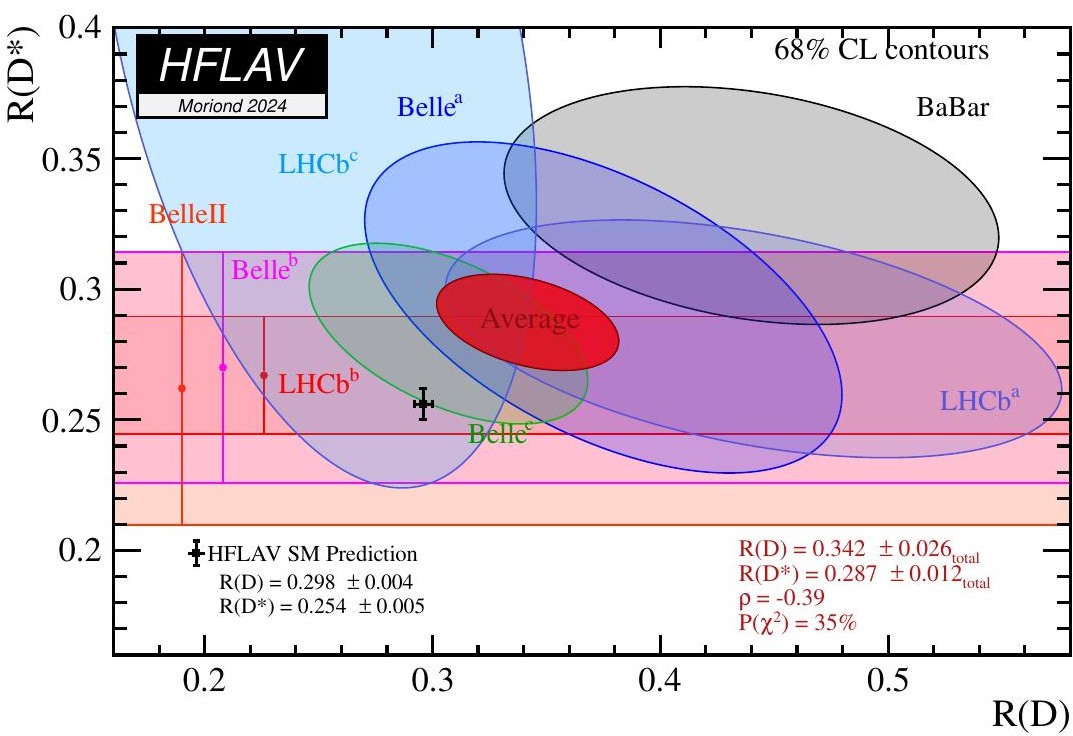
The LHCb group at Imperial has activities towards these lepton universality tests. We made the first observation of a Cabibbo suppressed semileptonic decay to a final state with two protons - paving the way for a novel universality test with a b→u quark transisition. This complements the current work targeting decays with b→c decays.
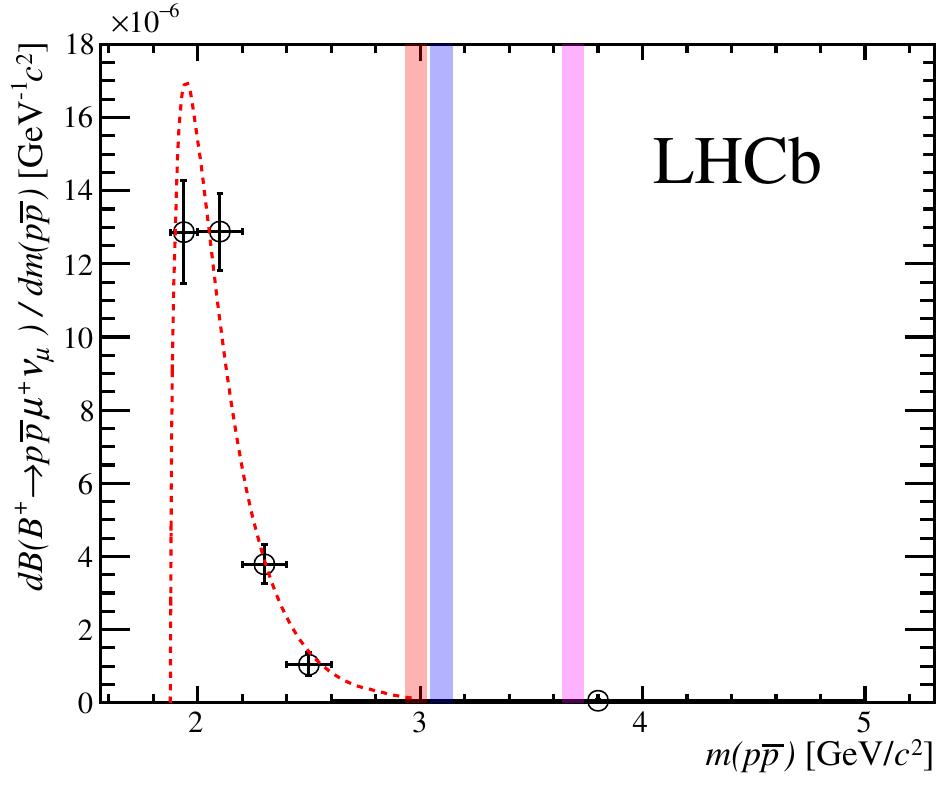
We are also studying semileptonic decays to final states with charm hadrons in excited states, the so-called D** states. Although these are poorly understood they pose a significant and irreducible background to all the current lepton universality measurements and in many instances are the source of the dominant systematic uncertainties. They also have significant interest in their own right as the different spin-parity states are sensitive to a variety of Lorentz structures and so they may be used to disentangle the form of any new physics. The LHCb group at Imperial is carrying out a comprehensive study of such decays, including differentially in the di-lepton invariant mass, in order to more accurately establish their branching fractions and help to discern the underlying form-factors.
gτ-2 Measurements
Measurements of magnetic moments offer exceptional sensitivity to beyond-the-Standard-Model (BSM) physics. In quantum electrodynamics (QED), the lowest order calculation using the Dirac equation predicts a gyromagnetic factor g = 2 precisely. Taking into account of higher orders corrections, the electron anomalous magnetic moment, a = (g−2)/2, is one of the most precisely measured quantities in physics and is in remarkable agreement with QED predictions. The g−2 of the muon has also been measured with high precision but shows a persistent discrepancy with certain theoretical predictions. By contrast, however, the tau lepton’s g–2 suffers from a lack of precision, given that its short lifetime makes direct measurements very challenging. If new-physics effects scale with the squared lepton mass mℓ2, deviations from QED predictions in this measurement would be about 280 times larger than in the muon g–2 measurement.
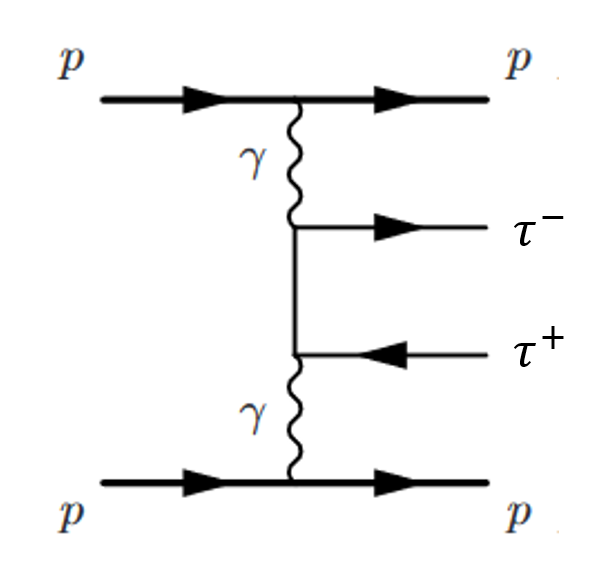
Imperial LHCb group works on measuring such a quantity via the exclusive production of tau–lepton pairs created in photon–photon collisions, where the value of g−2 is observed from the photon-lepton vertices. Passing each other at relativistic velocities in the LHC beampipe, the charged particles generate intense electromagnetic fields, where photon–photon collisions happen while keeping the incident particles intact. The final-state particles can be produced exclusively, with no other particles coming from the same production vertex, and thus offer a clean observation of tau decay products. The group is now working with the entire dataset from LHCb to collect these events for a high precision measurement of the g−2 quantity.
Vub Measurement
Vub is one of the CKM matrix elements in the Standard Model, describing the probability of a transition from a beauty quark to an up quark. It can be measured from the charmless leptonic decay b → uℓν, where a beauty quark decays into an up quark, a lepton, and a neutrino. Alternatively, it can also be extracted from the leptonic decay B → τν, where the beauty quark annihilates with the anti-up quark to produce a tau lepton and a neutrino.
The LHCb group at Imperial has proposed a new method to measure Vub , where a charm-strange meson is required in the final state to mitigate the large hadronic background, alongside the emission of a real photon. This process has only one α suppression and fewer tracks to reconstruct, providing a relatively clean channel for measurement.
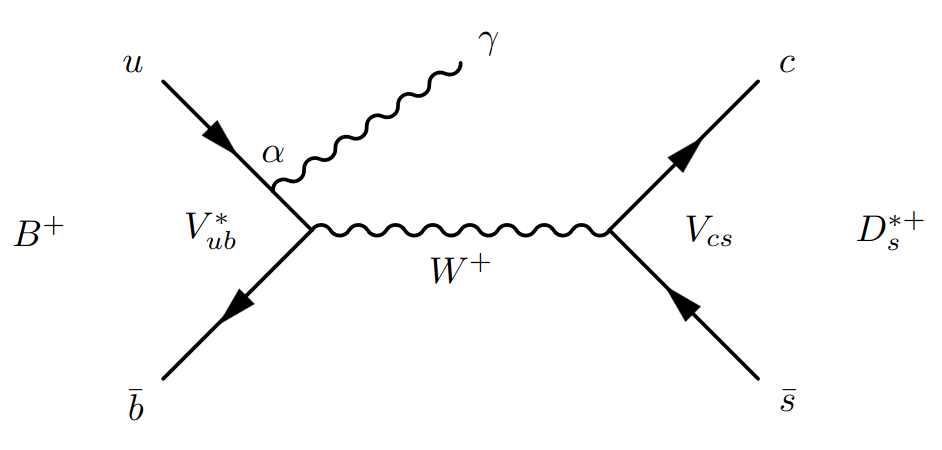
According to angular momentum conservation, the final state D meson must be an excited state, D*s, which promptly decays into a Ds and a soft photon. Since only one Ds track is available, the B meson vertex cannot be reconstructed. Additionally, two photons must be reconstructed: a soft photon from the D*s decay and a hard photon from the B decay. These are some of the challenges associated with this channel. We are currently working on this measurement using all available LHCb data.