Experiment overview
- Motivation
- Aluminum monofluoride (AlF) - an excellent candidate molecule for laser cooling
- Contact us
- Using lasers to reduce the thermal motion of particles
- Developing new deep ultraviolet lasers for applications in Quantum Technology
Why cool atoms and molecules to low temperatures?
Our experiments start by producing a molecular beam of the species of interest. When a gas expands through a small orifice into an evacuated chamber at very low pressure, it forms a directed beam of particles moving at about 100 m/s. The density of the molecules is typically very low and there are only a few collisions between them. This technique has been used for over 100 years to study atoms and molecules and the interaction of the particles with electric, magnetic and electromagnetic fields. We can slow the beam to a standstill using electric fields or precisely tuned lasers. The molecules can then be trapped and cooled to very low temperatures of a few millionths of a degree above absolute zero. At such a low temperature the molecules move with a speed of about 1 cm/s. This allows us to study the molecules, the interactions between the molecules and the interaction of light with the molecules with incredible precision and use them as a new resource for applications in Quantum Technology.
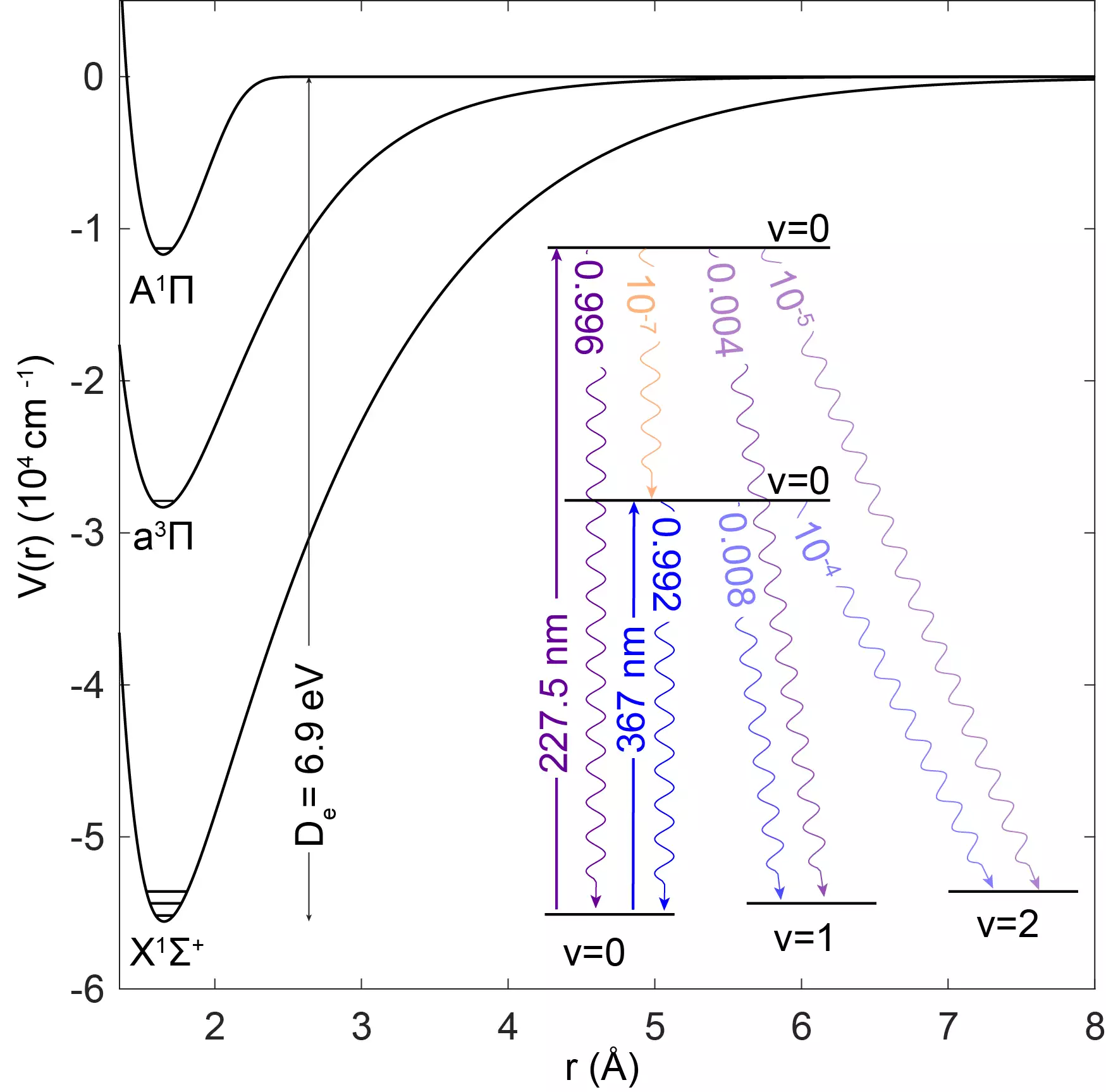
Most applications of ultracold molecules require and asymmetric charge distribution, i.e., an electric dipole moment and therefore heteronuclear, polar molecules. To reach a high density it is useful to start with a stable molecule such as NO or CO, but these molecules are very difficult to laser cool, or do not have a dipole moment. AlF cannot be purchased in a gas bottle, but can be produced with a very high efficiency using the reaction 2Al + AlF3 → 3 AlF at about 650 ℃. It can be laser-cooled to very low temperatures and has a large electric dipole moment of 1.5 Debye in the electronic ground state.
It has a very strong transition in the UV (excited state lifetime of 2 ns) and the probability of exciting a vibrational mode is extremely low. This allows exerting a large optical force which results in an exceptionally large capture velocity for the MOT, only limited by the currently available laser power in the UV. This means that we can build the MOT close to the molecular source, where the density is still very high and capture a large fraction of the molecular beam. For molecule standards the MOT is relatively simple. There is no need to modulate the laser frequency to address unresolved hyperfine structure in the electronic ground-state and polarization modulation is not required to destabilize dark hyperfine states.
AlF has a metastable (a3Π) state that lies in between the ground and first excited singlet states. The transition to this state is nominally forbidden, but becomes weakly allowed due to spin-orbit mixing with the singlet states. Laser cooling on such weakly-allowed intercombination lines are commonly used to cool atoms to a very low temperature at a very high density.
This project is funded by the ERC Starting Grant - CoMoFun
Enquiries can be directed to Dr Stefan Truppe - s.truppe@imperial.ac.uk
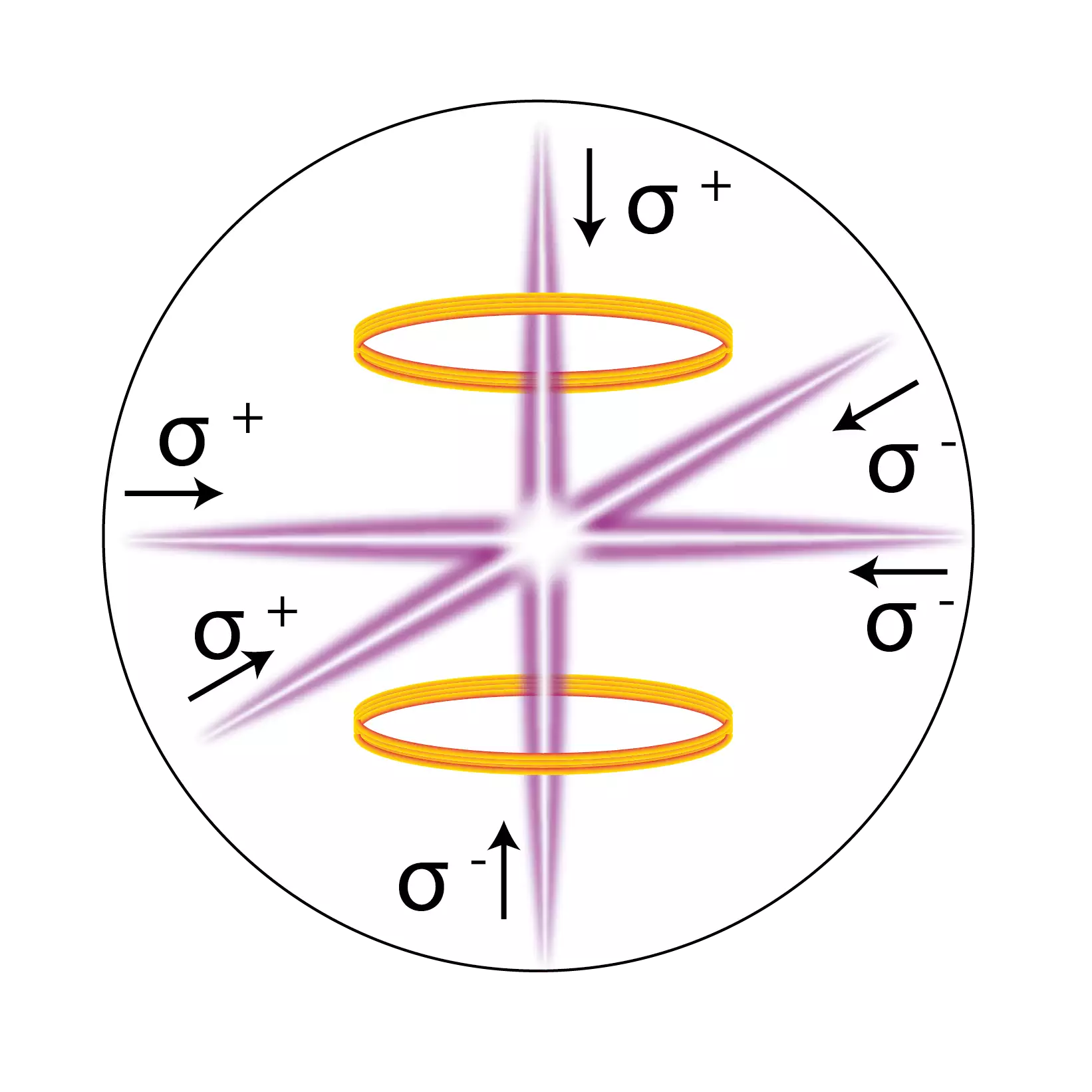
Laser-cooling atoms have paved the way
For over 30 years atoms have been trapped and cooled in a magneto-optical trap (MOT) using a combination of precisely tuned lasers and magnetic fields. The MOT has enabled the invention of precise instruments, such as atomic clocks, precise GPS, magnetometers, gravimeters and accelerometers. It has also enabled new fundamental research with unprecedented precision and the study of matter dominated by quantum effects.
Molecules offer even more. They are not spherically symmetric and can be oriented, they can rotate, vibrate, and have an electric and/or a magnetic dipole moment. Precisely controlled molecules can be used to test the most fundamental models in physics, to study new phases of matter, to model complex quantum systems and can serve as elements of a scalable quantum processor. However, the higher complexity makes it more difficult to cool the molecules. The MOT relies on the continuous scattering of photons, i.e. excitation of the particle followed by spontaneous emission. Atoms can scatter millions of photons by choosing a closed optical cycle between two electronic states.
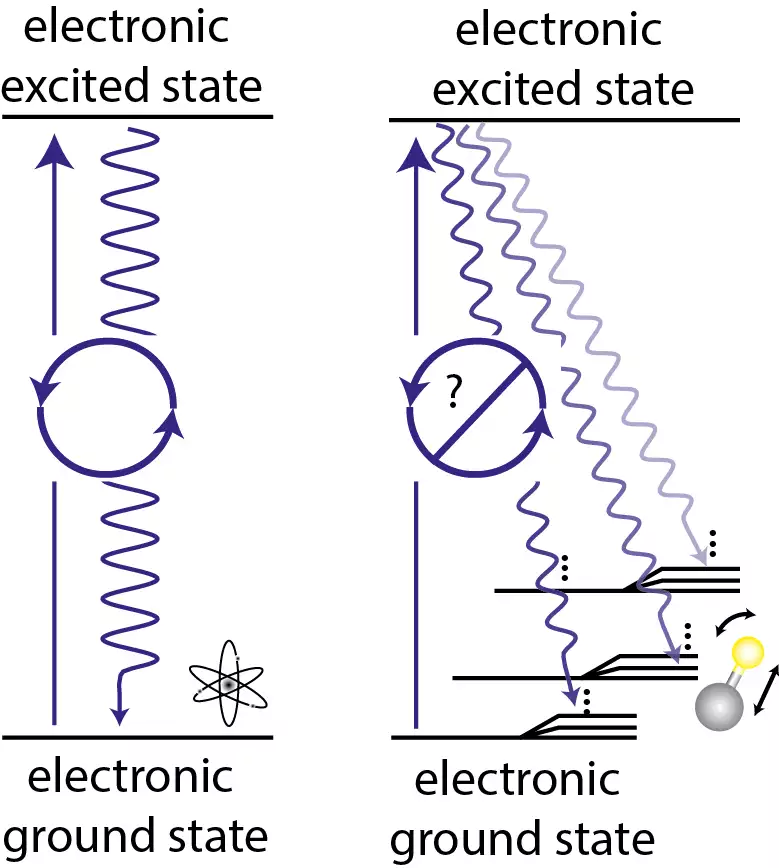
Molecules are more difficult to laser-cool because the laser can excite rotational and vibrational degrees of freedom. After a few photon-scattering events the molecule will end up in a "dark" state that is not coupled to the laser light and the optical cycle is broken.
The spontaneous emission to dark rotational states can be prevented by using quantum mechanical selection rules. However, there are no selection rules to prevent the emission to dark vibrational states. By selecting a molecule whose bond is not affected by promoting an electron into a higher orbital with a laser it is possible to minimize the probability of exciting vibrations. The periodic table offers 6903 hetero-nuclear diatomic molecules, so there are plenty of options to choose from. Currently, our favourite molecule for laser cooling is aluminium monofluoride (AlF). With only one laser to address a small 'leak' to a vibrationally excited state, we can scatter over 10000 photons, enough to slow, trap and cool the molecules.
We develop new lasers in the deep ultraviolet for applications in Quantum Technology. This project is funded by the Horizon Europe Quantum Flagship Project UVQuanT
Overview: UVQuanT is a groundbreaking project focused on developing new deep ultraviolet (DUV) laser systems to advance quantum technology. These innovative lasers will support applications like atomic clocks and quantum sensors, overcoming current limitations and creating new research and industrial opportunities.
Mission: Our goal is to create cost-effective, high-performance lasers for the 230-185 nm range. By combining the expertise of leading industrial and academic partners, UVQuanT aims to close the technology gap between visible and deep UV light, bringing advanced laser technology to a wider audience.
Goals:
- Develop stable, powerful DUV lasers at a lower cost.
- Enable new quantum technology applications, such as optical clocks and quantum sensors.
- Strengthen the supply chain for laser technology in Europe.
Impact: UVQuanT will benefit various sectors, including the semiconductor industry, medicine, and environmental science. The project will provide new tools for detecting harmful substances and enhance research capabilities in quantum technology.
Key Highlights:
- Develop robust DUV laser systems below 240 nm.
- Introduce new applications for DUV lasers in quantum technology.
- Collaborate with leading institutions and integrate into existing quantum projects.
- Reduce costs and advance technology to support European leadership in photonics.
Partners: UVQuanT brings together top academic and industrial partners:
- Imperial
- Fritz-Haber-Institute of the Max Planck Society (Germany)
- University of Bonn (Germany)
- Università degli Studi di Firenze (Italy)
- Chalmers University of Technology (Sweden)
- Vexlum Ltd. (Finland)
- Agile Optic GmbH (Germany)
- Eksma Optics (Lithuania)
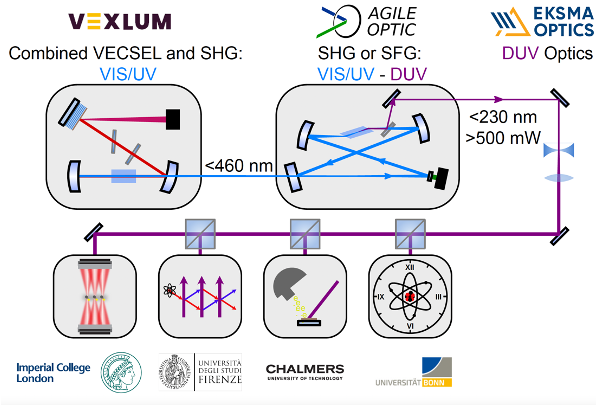